1. Introduction
2. Nasal spray
In recent years, administration through the nasal mucosa has been recognized as one of the modes of administration in which drugs can be rapidly and efficiently absorbed. There are many fine villi on the cells of the nasal mucosa, which greatly increases the effective area of ​​drug absorption. Due to the abundant blood vessels and lymphatic vessels under the mucosal cells, the drug can directly enter the systemic circulation after being absorbed through the mucosa. In addition, intranasal enzyme metabolism is far less than the gastrointestinal tract, therefore, nasal system is increasingly attention has been paid, including peptides and proteins study drugs. Among the many administration forms, the spray is a relatively common dosage form, and the liquid is atomized and ejected only by the power generated by the atomizing device by means of compressed air. Since the spray does not contain a propellant, there is no need to use a pressure-resistant container, and the field of application is now more and more extensive. During the nasal spray study, it is important to have two factors that influence the size distribution of the nasal spray, namely the drug formulation and the spray device. You can find out how laser diffraction technology can reflect these influencing factors through the following simulations.
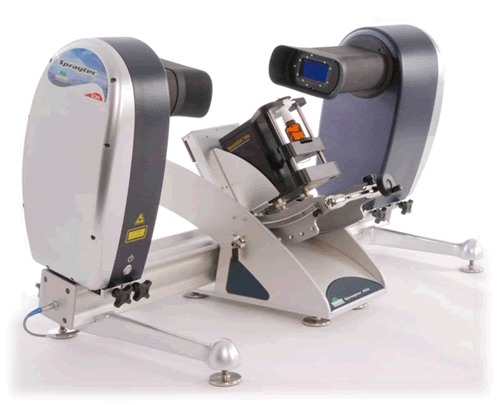
Figure 1. Malvern spray particle size analyzer for nasal spray particle size distribution
First, a brief introduction to laser diffraction technology to measure a nasal spray is a process. Figure 1 is a Malvern spray particle size analyzer. The devices erected at both ends are the transmitting end and the receiving end of the laser respectively. These devices can be freely moved to adjust the spatial position. The middle device is a nasal spray triggering device and is set as required. Different trigger pressures or trigger speeds (also useful for trigger time), and the spray angle can be adjusted so that the test parameters can be adjusted flexibly and quickly.
After the test is completed, the laser particle size analyzer will give the status of the entire injection process in real time. Figure 2 is a data of a nasal spray. The abscissa is time, the ordinate is the particle size, and several different color curves represent D10, D50, D90 and the injection concentration as a function of the injection time. The entire 0.16 second injection process can be divided into three stages, 0-0.02 seconds is the trigger stage, at this time the particle ejection is still unstable, the particle size is rapidly reduced, and the concentration is also rapidly reduced; 0.02-0.09 seconds is the stable stage, this The particle size distribution data tends to be stable; 0.09-0.16 seconds is the dissipation phase, at which time the particle size distribution becomes extremely unstable, and a large number of large particles appear. Laser diffraction technology not only gives a clear change process, but also gives the average particle size for the entire test process or for each stage. Figure 3 is a graph showing the average particle size distribution and particle size data for each stage.
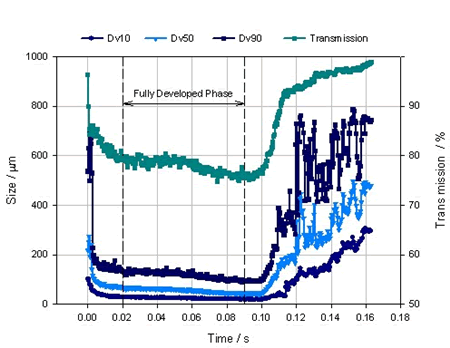
Figure 2. Nasal spray for a whole process
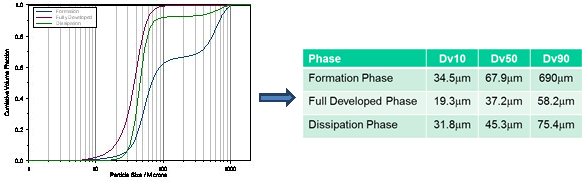
Figure 3. Separate particle size distribution and cumulative data for a three-stage nasal spray
It can also be seen from Fig. 3 that the average particle size in the initial stage is about 68 μm, and the particle size becomes smaller after stabilization to 37 μm, and the particle size in the dissipating stage is further increased to about 45 μm. In Fig. 4, the injection data of four consecutive times is given, so that not only the particle size change and the average particle size of each pass can be seen, but also the difference between the different times can be seen conveniently and quickly. Data changes and stability. Figure 5 is a particle size data for a spray formulation designed for 50 cycles throughout the spray cycle. It can be seen from the data that, except for the first-order particle size, the data is still relatively stable up to 60 times, and 41 of them may be due to the operation failure, resulting in a significantly larger spray particle size. This method of operation provides a good data base for the spray cycle and stability of the nasal spray and tank design.
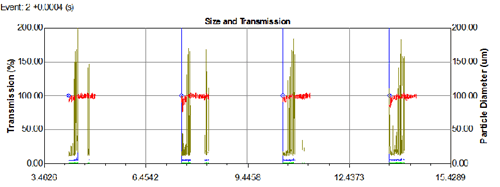
Figure 4. Spray data for 4 sprays of nasal spray
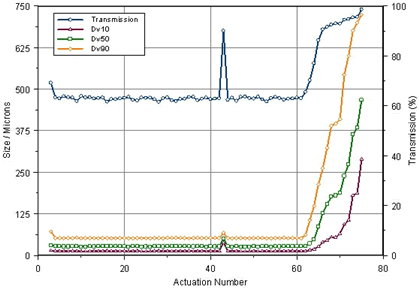
Figure 5. Particle size data for a 50-injection nasal spray throughout the spray cycle
In addition to the stability of the shots, it is also possible to observe the effect of different formulations, different jet pumps and different jet sizes on the spray size. Figure 6 shows the droplet size of the same nasal spray formulation under different jet pump conditions.
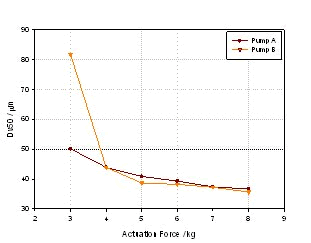
Figure 6. Effect of spray size on the same nasal spray formulation under two different pump conditions
It can be seen from the figure that the droplet size is significantly reduced with the increase of the trigger pressure of the two pumps, but in contrast, the B pump is not sensitive to pressure, while the A pump is at a lower pressure. As the pressure changes, the particle size will change greatly. These are the issues that must be considered when designing and selecting the pump.
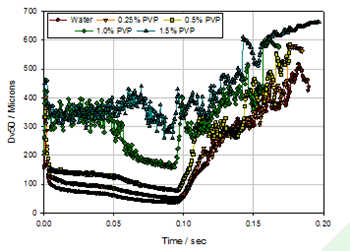
Figure 7. Effect of different concentrations of PVP on spray particle size (A pump)
Of course, the drug formulation also has a large effect on the particle size of the spray. The results can be observed through a simulation experiment. Under the same apparatus and the same pump speed conditions (40 mm/s), different concentrations of PVP aqueous solution were used to observe the atomization effect, and the PVP concentrations were 0, 0.25%, 0.5%, 1.0%, and 1.5%, respectively. Figure 7 shows the spray median particle size results for the five formulations. It can be seen that as the PVP concentration increases, the atomization particle size becomes larger and the atomization stabilization period becomes shorter and shorter, when PVP When the concentration reaches 1.5%, it is basically impossible to find a stable atomization state. The reason may be that as the concentration of PVP increases, the viscosity of the atomized liquid increases, resulting in a significant increase in the particle size of the atomized droplets . However, for the formulation of the same trend, we replaced the jet pump B, and the result is shown in Fig. 8. It can be seen that although the tendency of the particle size to increase with the increase of the PVP concentration has not changed, the spray stability is significantly increased, which also indicates that the shear force provided by the B pump completely overcomes the fluctuation caused by the increase in the viscosity of the atomized liquid. In order to further investigate the influencing factors affecting the particle size of the spray, a more fine nozzle was observed to observe the atomization effect while maintaining the experimental conditions of FIG. Figure 9 shows the results of atomized particle size at finer nozzles for PVP concentrations of 0, 0.5%, and 1.0%. It can be found that the particle size distribution of the atomized liquid is significantly smaller, especially at the 1.0% PVP concentration, and the atomic droplet diameter of the atomized droplet is reduced from 200 μm to 120 μm.
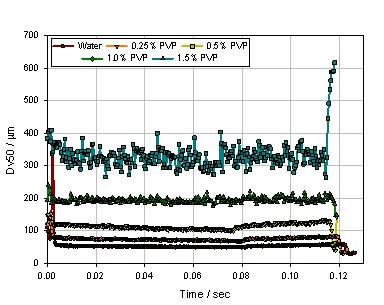
Figure 8. Effect of different concentrations of PVP on spray particle size (B pump)
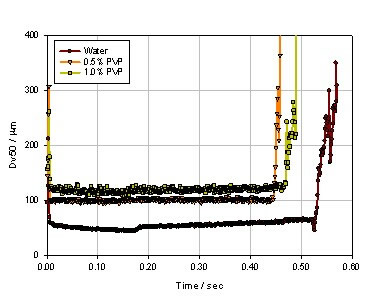
Figure 9. Experimental results for nozzles with smaller apertures (B pump)
3. Nebulizer spray
A spray refers to a dosage form in which a drug solution is driven by compressed air to reach a dispersed drug through a spray hole. Although it has many deficiencies, such as large size and inconvenient carrying, complete atomization system is expensive, can only deliver one dose, etc., but its advantages are also obvious, such as no need for propellant, tank container without pressure, generally taken The water-based formula is supplemented with fixed excipients. At the same time, for drugs with higher inhalation doses (such as Novartis's 300mg tobramycin), spray atomization delivery also has obvious advantages. In addition, tidal breathing can be used. Currently, sprays are widely used in hospital emergency rooms, especially for children with asthma or chronic obstructive pulmonary disease and elderly patients. Sprays are also a very important formulation for formulating and atomizing. In other words, only a good formula with a suitable atomization method can make a good spray. Of course, due to the different breathing patterns, it may also affect the particle size of the inhaled droplets. Therefore, the atomization formula, atomization method, and breathing mode must be considered during the research.
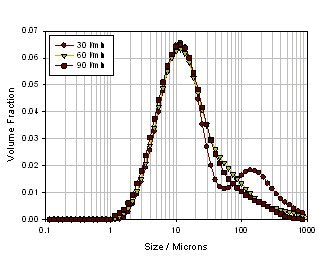
Figure 10 is a schematic illustration of a Malvern spray particle size test spray formulation. On both sides are the transmitting and receiving ends of the laser, and in the middle is a suction-type sample cell that simulates the human respiratory tract, while the white curved tube above is the USP artificial throat, and the suction-type sample cell is connected to the pump or breathing apparatus. This liquid mist enters the laser test area through the upper artificial throat and is pumped away by inhaling the sample cell. Figure 11 is a particle size distribution of continuous liquid mist atomization. The abscissa is time and the ordinate is particle size. The curves of the three colors are D10, D50 and D90 of the droplet size. It can be seen that the particle size distribution of the droplets is relatively stable over an atomization time of up to 10 minutes. Various factors affecting the atomized particle size can be understood by the following experiments. There are many ways to atomize liquid mist, such as common spray atomization, vibration atomization or ultrasonic atomization. Each atomization has its own advantages and disadvantages. Among them, spray atomization is a common method. The main principle is that the liquid is carried through a narrow nozzle by a certain speed of compressed air. At this time, the flow rate of compressed air has a great influence on the atomization effect. . Figure 12 shows the atomized particle size results for the same nozzle at different air flow rates . It can be seen from the figure that as the air flow rate increases, the particle size parameters D10, D50 and D90 of the atomized droplets decrease, and when the flow rate reaches 11 L/min, the atomized particle size reaches a minimum, followed by air. The flow rate is further increased, and the atomized particle size is rather large, which may be caused by a flow rate that is too large to cause a portion of the large droplets to pass over the baffle.
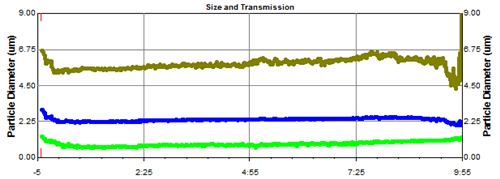
Figure 11. Continuous nebulizer atomization particle size test results
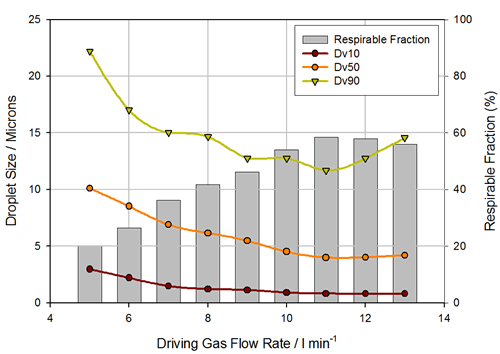
At the same time, the Malvern spray particle size analyzer can be used in conjunction with a breathing simulator to further investigate atomization. Figure 13 shows the atomization particle size of an atomization system in sinusoidal breathing mode. As the inhalation rate gradually increases, the atomized droplet concentration increases rapidly and tends to be stable, while the atomized droplet size is rapidly Decrease and then slowly increase, and as the inhalation rate gradually becomes smaller, the concentration of the nebulized liquid rapidly decays and the particle size of the nebulized liquid begins to increase significantly and is unstable, and this data also well reflects the changes that occur during the breathing process. Of course, you can also change the way you breathe, such as maintaining the same formula and tubing structure, increasing the respiratory rate, and observing the effect of breathing pattern on the atomized particle size (Figure 14). It can be seen from the figure that as the respiratory rate increases, the inhalation time also decreases accordingly, and the flow rate of the inhaled droplets also increases, and the droplet size decreases remarkably.
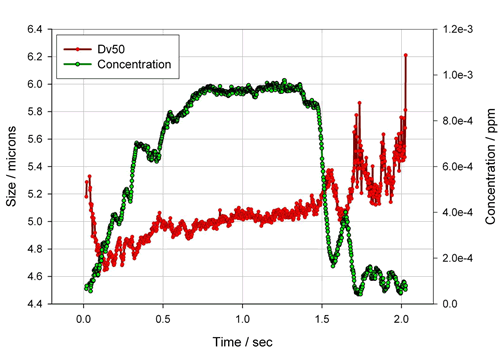
Figure 13. Atomization granularity results for an atomizing system in sinusoidal breathing mode
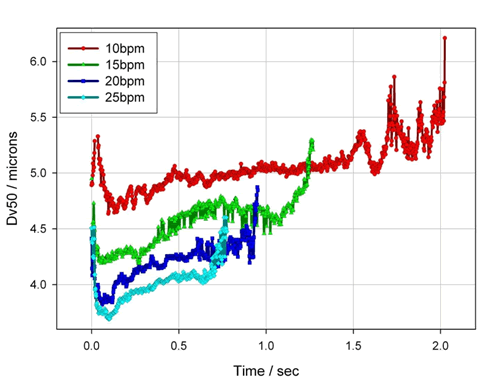
Figure 14. Atomized droplet size results at different respiratory frequencies
In addition to the breathing mode, the formulation of the mist also has a significant effect on the atomized particle size. Figure 15 shows the atomized particle size results for three different concentrations of PVP solution. It can be seen that as the PVP is added and the concentration is increased, the atomized particle size is significantly increased, mainly because the addition of PVP increases the viscosity of the atomized liquid. At the same time, Fig. 16 shows the change of the mist inhalation concentration of the above three kinds of atomized liquids during the inhalation process. As can be seen from the figure, as the PVP is added and the concentration is increased, the inhalation concentration is significantly reduced, which means that a higher viscosity atomized liquid may require a longer inhalation time in order to achieve the same delivered dose. .
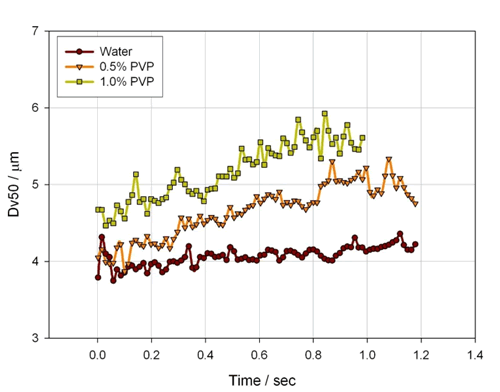
Figure 15. Atomized particle size results for different concentrations of PVP solution
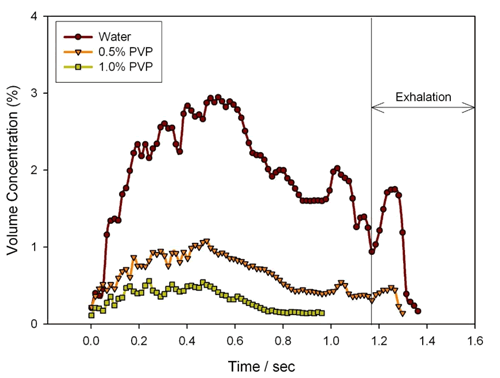
Figure 16. Results of aerosolized concentrations of different concentrations of PVP solution
4. DPI dry powder inhaler
Dry powder inhaler (DPI), also known as inhalation powder, is a new formulation developed on the basis of quantitative inhalation aerosol combined with powder delivery process. It is a method in which the micronized drug is mixed with the carrier alone or through a special drug delivery device, and the drug is dispersed into a mist into the respiratory tract by a patient's active inhalation, thereby achieving local or systemic administration. Dry powder inhalers have their own distinctive features: for example, there is no need for Freon propellant, no air pollution problems; no solvent solvents such as alcohol and preservatives, which reduce the irritation to the throat and are also easier to preserve; The dose that can be carried is higher; the solid dosage form is especially suitable for polypeptides and protein drugs. However, dry powder inhalers do not need to consider the problem of dissolution and suspension, but because of the easy agglomeration between the powder particles, the factors of coating or interaction between the active ingredient and the auxiliary carrier must also be considered in detail, which has more to the inhalation device. High requirements, which means that it must be a suitable active ingredient and carrier, control the appropriate particle size, and with a suitable inhalation device, in order to achieve a stable and safe dose delivery.
To further illustrate this problem, different inhalation devices were used to observe the atomization effect through two different drugs. Two of the powder drugs were salbutamol and budesonide, and Table 1 shows the proportion of atomized fine particles. It can be seen that the dispersion effect of the same material in different inhalation devices is very different. For example, the proportion of fine particles of budesonide can be changed from 14% to 63%. However, if the molecular surface energy of budesonide is more than five times that of salbutamol from the viewpoint of powder physical properties, it means that the particles of dispersed budesonide are much more difficult than that of salbutamol. But seeing the end result, on the contrary, the budesonide powder has more fine particles dispersed, which further indicates that the powder inhalation dispersion is not simply carried out according to the laws of its physical properties, so if it is to be dried powder inhalation preparation For research and development, it is necessary to consider the powder formulation and the inhalation device at the same time.
Table 1. Fine particle ratio of two powders under different inhalation devices
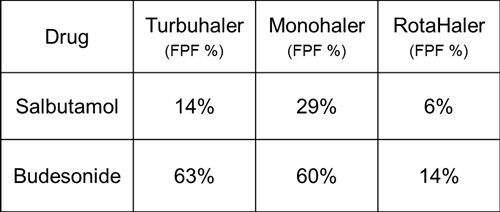
Next, a small experiment was conducted to see how the powder formulation process, inhalation device, and inhalation rate affect the atomization effect. Three kinds of powders are selected (see Table 2). The first one is ordinary micronized lactose powder, the second is micronized lactose added with 5% MgSt, and processed by laboratory common mixing equipment. Three of the same micronized lactose were added with 5% MgSt, but a high-intensity mixing device was used (this technique was developed by Vectura). Since magnesium stearate itself acts as an amphoteric substance, it can form a coating structure on micronized lactose, thereby reducing the agglomeration of lactose, but at the same time the mixing mode and efficiency will greatly affect the efficiency and uniformity of lactose encapsulation. It also directly leads to the complexity of powder delivery. Figure 17 shows the particle size distribution of pure micronized lactose at different inhalation rates. It can be seen from the figure that as the inhalation rate increases, the particle size of the particles decreases significantly, indicating that although the lactose itself is relatively small. of. However, since the fine particles have a strong agglomeration effect, as the inhalation rate increases, the shearing force increases, resulting in smaller and smaller particles, but the agglomeration is still obvious. FIG 18 shows normal mixing lactose + magnesium stearate, particle size of the suction body at different rates. Compared with pure lactose, the particle size of the particles is firstly reduced at a low inhalation rate, especially in the case of large particles, which indicates that the encapsulation of magnesium stearate reduces the agglomeration of lactose to some extent. However, as the inhalation rate increases, the particle size changes are not obvious, and the agglomeration is still very obvious, which means that the magnesium stearate is not evenly packaged, in other words, it does not form a wrap on the surface of a single lactose particle, but multiple The lactose agglomerated particles are encapsulated. Thus, these large encapsulated particles do not disperse as the inhalation rate increases, thus causing the particle size to be larger at the high flow rate than the pure lactose. However, if the processing method is improved, the dispersion uniformity and the encapsulation efficiency of magnesium stearate are improved, and the encapsulation of the individual lactose particles is achieved, the dispersed particle size can be greatly improved. Figure 19 shows the particle size of the powder in a high-energy mixing mode under different inhalation conditions. It can be seen that the dispersed particle size is greatly reduced, basically below 20 microns, and its particle size distribution is not for the inhalation rate. Sensitive, all of which indicate a significant increase in the efficiency and uniformity of lactose encapsulation.
Table 2. Powders of three different formulations and processing techniques
Powder formula | Processing methods |
Micronized lactose | no |
Micronized lactose + 5% MgSt | Conventional laboratory mixing equipment |
Micronized lactose + 5% MgSt | High-intensity mixing equipment |
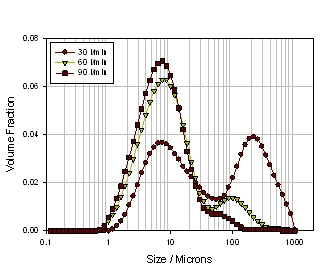
Figure 17. Particle size distribution of pure micronized lactose at different inhalation rates
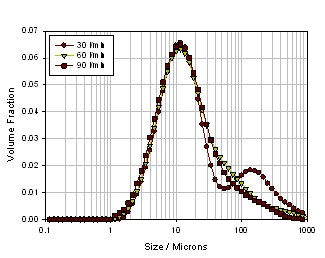
Figure 18. Particle size distribution of commonly mixed lactose + magnesium stearate powder at different inhalation rates
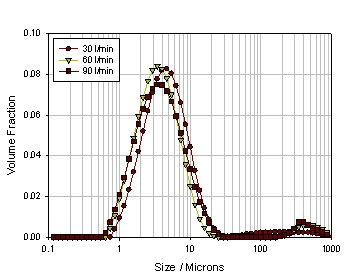
Figure 19. Size distribution of high-energy mixed lactose + magnesium stearate powder at different inhalation rates
5. Laser Diffraction & Impactor Connection
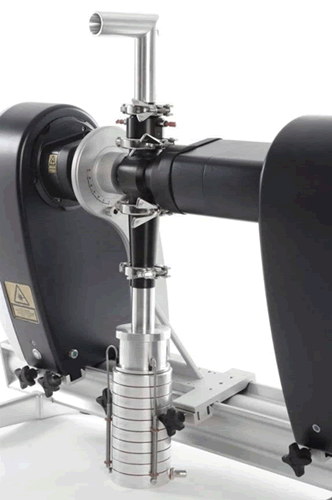
Figure 20. Laser Diffraction Particle Size Analyzer and Anderson Impactor Connection
In order to make the measurement conditions of laser diffraction consistent with the test conditions of the collision method, the laser particle size analyzer can also be connected with the relevant collider. Figure 20 is a schematic illustration of the Malvern spray particle size analyzer coupled to the Anderson striker, wherein the inhalation formulation enters the inhalation sample cell through the upper artificial throat for particle size detection and then enters the impactor through the lower interface. Since the matching of test conditions is greatly improved in the same path, laser diffraction as a non-destructive testing technology itself does not have any influence on droplets and droplets in the path, thus greatly expanding its applicability.
6. Summary
At present, inhalation preparations are receiving more and more attention from everyone. Whether it is aerosol, liquid mist or powder mist, no matter what form, particle size detection is undoubtedly an indispensable part of in vitro testing. The current process of pharmaceutical R&D is actually a process of racing against time, so how to quickly screen and screen a large number of recipes, spray devices and test conditions during development is critical. The laser diffraction technology has the characteristics of fast and non-destructive, and the result is very strong. It can quickly provide a large number of data related to particle size detection, which provides a solid guarantee for the development and production of inhaled preparations.
(Author: Li Xuebing, Malvern Instruments Ltd. UK size grain shape Technical Experts)
We're Professional Supplier Extract Powder manufacturers and suppliers in China specialized in providing high-quality products at low price. We warmly welcome you to buy or wholesale bulk Supplier Extract Powder for sale here from our factory. For a free sample, contact us now.
Supplier Extract Powder,Supplier Extract ,Supplier Powder Manufacturer in China
Shaanxi Kang New Pharmaceutical co., Ltd. , https://www.bodybuildingoil.com